What are some of the materials traditionally used in fusion reactors as ‘breeding blankets’, and what is their function?
Fusion reactors could be a powerful means of generating clean electricity, and currently, and several potential designs are being explored. In a fusion reactor, the fusion of two nuclear atoms releases massive amounts of energy. This energy is trapped as heat in a “breeding blanket (BB),” for example a liquid lithium alloy, surrounding the reactor core. This heat is then used to run a turbine and generate electricity. The BB also has an essential function of fusion fuel breeding for creating a closed fuel cycle for the endless operation of the reactors without fuel depletion.
What are ‘liquid metal fuel breeders’?
The liquid metal fuel breeders of fusion reactors are called “liquid breeders”, and the BB with liquid breeders is called a “liquid blanket”. This liquid blanket is a high-performance component of fusion reactors since it can produce heat and fusion fuel at the same time.
The operation of the liquid blanket at extremely high temperatures over 1100 K serves the function of producing hydrogen from water, which is a promising technology to realize a carbon-neutral society. This is possible because the BB heats up to over 1100 K by absorbing the energy from the fusion reaction. At such temperatures, there is the risk of structural materials in contact with the BB corroding, compromising the safety and stability of the reactors. It is thus necessary to find structural materials that are chemically compatible with the BB materials at these temperatures.
What temperatures are you able to demonstrate corrosion resistance up until?
Liquid metals are metals or metal alloys liquid at a working temperature. Liquid metals can dissolve some metal elements of structural materials (e.g., iron (Fe), chromium (Cr), and nickel (Ni)) due to the reactions of dissolution and alloying. The surface of the structural material can become porous due to the depletion of metal elements as a result of dissolution corrosion when the structural materials are exposed to liquid metals at high temperatures for a long time.
Figure 2 introduces some examples of steels corroded in liquid metals. The steels were significantly corroded at around 823 K from 250 hours to 1000 hours due to the depletion of metal elements from their surface. The corrosion intensity is more significant at higher temperatures. Therefore, it was not expected that any structural materials could survive in liquid LiPb at 900 °C.
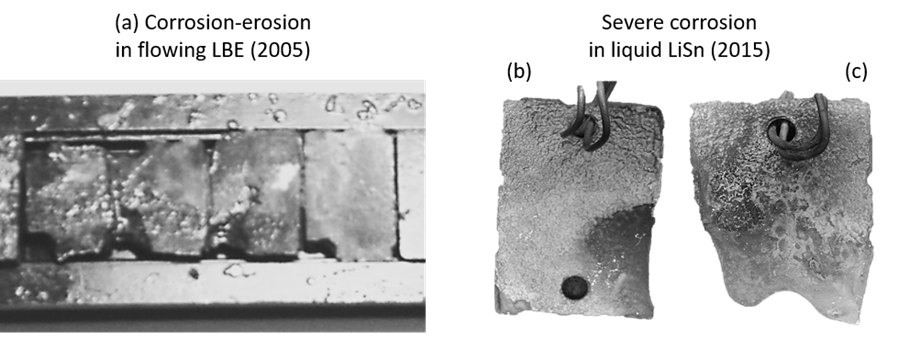
Your research has explored the corrosion resistance of some structural materials at much higher temperatures. What materials were these, and why were they selected for your research?
Our team of scientists from the Tokyo Institute of Technology (Tokyo Tech), National Institutes of Quantum Science and Technology, and Yokohama National University, Japan, have demonstrated compatibility at much higher temperatures. Our recent study makes clear the nuances of the corrosion resistance mechanism of CVD-SiC and FeCrAl alloys in liquid LiPb up to 1173 K. We recently reported the research findings in an article in Corrosion Science, which is an authoritative journal on material compatibility.
Our team first synthesized high-purity LiPb by melting and mixing granules of Li and Pb in an apparatus under vacuum conditions, as shown in Fig. 3. They then heated the alloy to the aforementioned temperatures, at which point it was liquified. Samples of CVD-SiC and two variants of the FeCrAl alloy—with and without pre-oxidation treatment to form an α-Al2O3 surface layer—were placed in this liquid LiPb for 250 hours for corrosion testing. Prof. Kondo observed that “An interesting finding is that, contrary to previous literature, oxidation pre-treatment to form an α-Al2O3 layer did not provide corrosion resistance beyond 1023 K.”
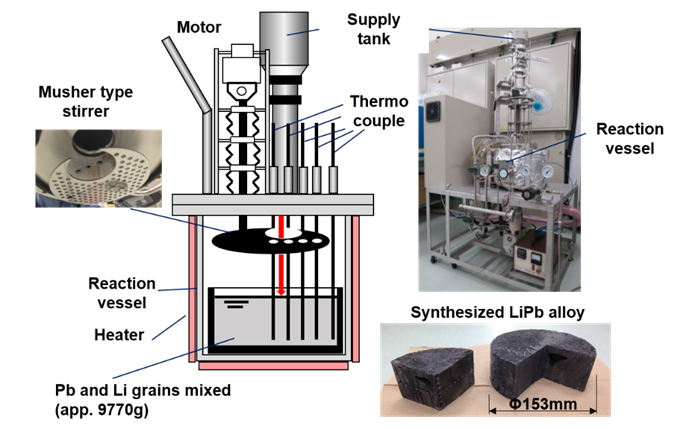
Cross-sections of the retrieved samples showed that CVD-SiC reacted with impurities in the LiPb alloy to form a layer of complex oxides, which then provided it with corrosion resistance. The untreated FeCrAl alloy formed a layer of the oxide γ-LiAlO2 upon reaction with LiPb, which then acted as an anti-corrosion barrier. In the case of the pre-treated FeCrAl, the α-Al2O3 surface layer provided corrosion resistance at 873 K but transformed into γ-LiAlO2 at 1173 K, and it was γ-LiAlO2 that then provided corrosion resistance.
The insights from these findings are expected to prove useful when designing and choosing new structural materials for fusion reactors, which could pave the way for a greener economy.
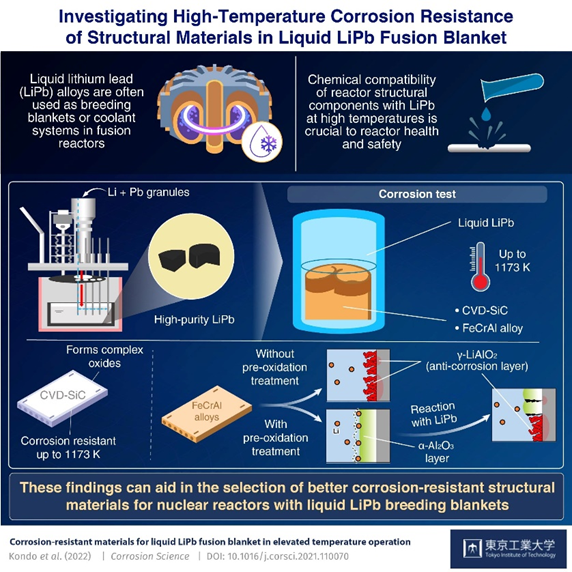
How significant do you believe fusion reactors can be to the clean energy revolution?
Fusion reactors can provide a huge amount of energy without CO2 emissions. The fusion fuels are deuterium and tritium. High energy neutrons produced by fusion reaction can breed tritium according to the transmutation of Li to tritium in the liquid blanket. Deuterium and lithium can be recovered from seawater, which makes them abundant. Therefore, fusion fuels are virtually inexhaustible. Thus the fusion reactor is a promising technology that can contribute to the sustainable development of human society based on carbon neutrality.
How important will your research be toward efforts to scale up the use of fusion reactors for clean energy production?
We clarified that FeCrAl alloys could be chemically compatible with liquid metal up to 1173 K. The fusion reactor can produce hydrogen from water and biomass when the liquid blanket can be operated at 1173 K. Thus, fusion reactors can provide not only electricity but also hydrogen for the future of society.
Source : Azom | Avoiding High-Temperature Material Corrosion in Fusion Reactors (azom.com)
No Responses